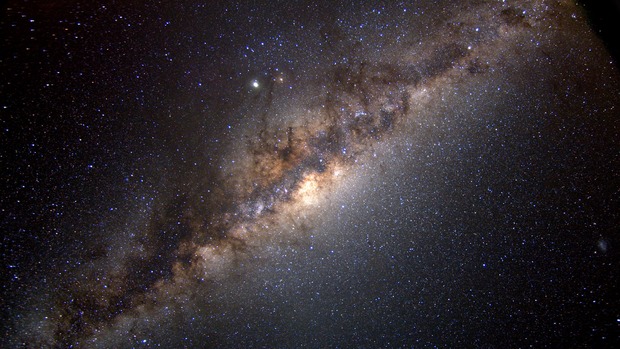
Our Milky Way galaxy. (Credit: NASA/S. Brunier)
Facts
Comprising the world's largest precision optical instruments and utilizing one of the world's largest vacuum systems, LIGO is a marvel of engineering and human ingenuity. Read on for some quick facts about LIGO, its past, and its exciting future.
Ever Changing and Improving Detectors
LIGO was funded by the US National Science Foundation (NSF) in the early 1990's when much of the technology required for LIGO's success did not yet exist. Although the NSF considered LIGO to be a "high risk, high reward" project from the start (they knew the chance of failure was high, but the chance of revolutionary discovery far outweighed the risk), they committed for the long haul with the knowledge that it would likely take decades of technological advancement before LIGO had any hope of seeing success.
Indeed, LIGO's instruments started off as modest prototypes, but over time, as technology and our knowledge of engineering have improved, they have grown exponentially both in scale and sophistication. In their quest to achieve what many had believed to be unachievable, LIGO's engineers and global collaborators have now worked together over more than three decades to improve the instruments and continually push the envelope of what is quite literally physically possible with current technology. As technology itself evolves, so too does LIGO, and with each step in its evolution over time, LIGO's instruments and others like it around the world have opened the field of gravitational wave astronomy. Today, we know that the enormous risk the NSF took paid off, and hundreds of scientists (and the interested public alike!) around the world are now reaping the rewards of LIGO's incredible journey from impossible dream to ground-breaking discovery.
To learn more about how LIGO's instruments have changed over time, visit Our Evolving Detectors.
LIGO and Cutting Edge Discovery Science
LIGO is one of the most sophisticated scientific instruments ever built. LIGO's detections are providing physicists with the means to answer key scientific questions, such as:
- What are the properties of gravitational waves?
- Is general relativity the correct theory of gravity?
- Is general relativity still valid under strong-gravity conditions?
- Are nature's black holes the black holes of general relativity?
- How does matter behave under extremes of density?
- What happens when a massive star collapses?
- How do compact binary stars form and evolve, and what can they tell us about the history of star formation rates in the Universe?
For more information on LIGO's impact on the broader scientific community, visit LIGO's Impact on Science.
LIGO's Extreme Engineering
LIGO exemplifies extreme engineering and technology. LIGO consists of:
- Two L-shaped detectors with 4 km long vacuum chambers...
- situated 3000 kilometers apart operating in unison...
- to measure a motion 10,000 times smaller than an atomic nucleus (the smallest measurement ever attempted by science)...
- caused by the most violent and cataclysmic events in the Universe...
- occurring tens-of-millions or billions of light years away!
A few of LIGO's most remarkable engineering facts are listed below.
Most sensitive: At its most sensitive state, LIGO will be able to detect a change in distance between its mirrors 1/10,000th the width of a proton! This is equivalent to measuring the distance to the nearest star (some 4.2 light years away) to an accuracy smaller than the width of a human hair.
World's third-largest vacuum chambers: Encapsulating 10,000 m3 (350,000 ft3), the air removed from each of LIGO’s vacuum chambers could inflate 2.5 million footballs or 1.8 million soccer balls. LIGO's vacuum volume is the third largest in the world, surpassed only by the Large Hadron Collider (LHC) in Switzerland (530,000 ft3), and NASA's "Space Simulation Vacuum Chamber" (800,000 ft3).
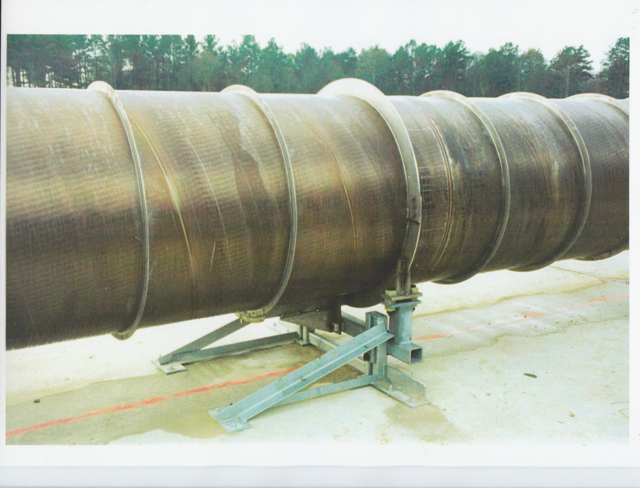
Exposed beam tube after assembly at LIGO Livingston. Steel support 'rings' are welded to the tube to prevent it from collapsing under the pressure of the atmosphere. (Credit: Caltech/MIT/LIGO Lab)
Ultra-high vacuum: LIGO's vacuum envelope may be the third largest by volume in the world, but they are the second largest "Ultra High" vacuum chambers (the first being the LHC). The pressure inside LIGO's vacuum tubes is one-billionth of an atmosphere (10-9 Torr)--in other words, one billionth the air pressure that you would encounter at sea level. It took 40 days to remove all 10,000 m3 (353,000 ft3) of air and other residual gases from each of LIGO’s vacuum tubes. This process was only conducted once, in 1998 at LIGO Hanford Observatory and in 1999 at LIGO Livingston. LIGO's vacuum tubes havecontinuously maintained this pressure ever since.
Air pressure on the vacuum tubes: 155-million kg (341-million pounds) of air press down on each 4 km length of vacuum tube. Remarkably, the steel tubes that hold all that air at bay are only 3 mm (0.12 inches) thick. To help prevent the tubes from being crushed by the atmosphere, they are encircled by steel support 'strips' every meter or so (photo at left).
Curvature of the Earth: LIGO’s arms are long enough that the curvature of the Earth was a factor in their construction. Over the 4 km length of each arm, the Earth curves away by nearly a meter! Precision concrete pouring of the path upon which the beam-tube is installed was required to counteract this curvature and ensure that when the laser beam leaves the ‘corner station’ (traveling in a straight line) it strikes the test mass/mirror at the end of each arm, and not a meter above it.