
Artist conception of the moment two neutron stars collide.
LIGO Celebrates One-Year Anniversary of Historic Binary Neutron Star Merger Detection
News Release • August 17, 2018
By Kimberly Burtnyk
Today LIGO commemorates the one-year anniversary of its most important discovery to-date: The detection of a merging pair of neutron stars, aka a BNS (binary neutron star) merger.
After a 130 million year journey, the gravitational waves generated by these exotic stars arrived at LIGO’s Hanford and Livingston detectors in the United States, and the Virgo detector in Italy on August 17th, 2017. Dubbed a ‘kilonova’ (a term coined in 2010 in a paper wherein it was theorized that a pair of merging neutron stars would emit light about 1000 times brighter than a classical nova), the detection also led to a massive explosion of multimessenger astronomy results gathered by astronomers from all around the globe. LIGO announced the discovery to the world with papers published on October 16th.
How important was this detection? Well, on the day of the announcement, 84 scientific papers were published about it.
Today, an internet search for “GW170817” will yield over 110,000 results, all related to this one event that captured the world scientific community’s attention (incidentally, a search for “GW150914”, LIGO’s first detection, yields a mere 80,000 hits).
What immediately followed the detection, and what continues to this day, is a level of scrutiny never before witnessed in the annals of scientific study.
Why all the Excitement?
Up until that day in August, LIGO’s detections had all been gravitational waves caused by merging black holes. While there’s no doubt that those discoveries have been monumental, the scope and magnitude of this discovery would prove unprecedented. The LIGO and Virgo detection has become probably the most widely studied astronomical event in human history. Within days, this object was being examined by nearly one-third of the world’s electromagnetic (EM) astronomers. The fact that EM astronomers were able to observe the phenomenon alongside GW astronomers is what truly elevated this event to history-making levels. LIGO’s previous detections of merging black holes did not result in such widespread study because, by their very nature, black holes are believed not to emit electromagnetic waves (i.e., light of any wavelength). No amount of searching by astronomers using telescopes designed to observe EM radiation has revealed anything. Only gravitational wave observatories like LIGO and Virgo can ‘observe’ black holes colliding.
Neutron stars are different, however. Unlike black holes, neutron stars are made up of actual matter, including copious amounts of neutrons (hence their moniker), and when you accelerate or slam matter together you get electromagnetic radiation (again, not something one expects to detect from colliding black holes).
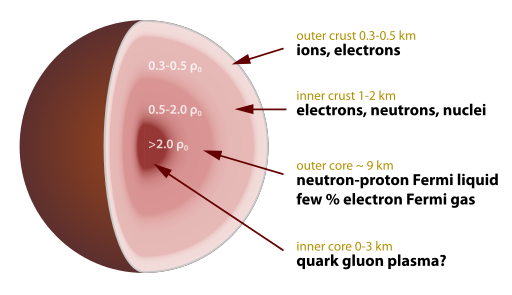
Basic anatomy of a neutron star. By Robert Schulze [CC BY-SA 3.0 (https://creativecommons.org/licenses/by-sa/3.0)
But like black holes, neutron stars are massive and compact enough to generate gravitational waves when they collide. This combination of properties (material composition and density) means that colliding neutron stars can emit both gravitational waves AND electromagnetic radiation: “light” in all its forms from gamma rays to radio waves. In fact, it was a burst of gamma rays, arriving 1.7 seconds after the gravitational waves, which alerted the broader astronomical community to something truly extraordinary and led to an unprecedented global effort to study the phenomenon.
Time-lapse video of all the observations of GW170817 that occurred around the world in the days following the event. Credit: The GROWTH Project Collaboration, NSF PIRE 1545949
What have we learned?
What immediately followed the detection, and what continues to this day, is a level of scrutiny never before witnessed in the annals of scientific study. So what have we learned (so far)? Below is a very short list of just some of the amazing outcomes and discoveries resulting from the worldwide effort to study this remarkable event.
- Our understanding of the origin of elements in the periodic table changed nearly overnight. Electromagnetic (EM) follow-up observations — possible because LIGO and Virgo's observations pin-pointed the location on the sky — have now confirmed a long-standing supposition that binary neutron star mergers generate a large fraction of the universe’s heavier-than-iron elements, including familiar ones like gold and platinum, to more exotic things such as thorium and uranium.
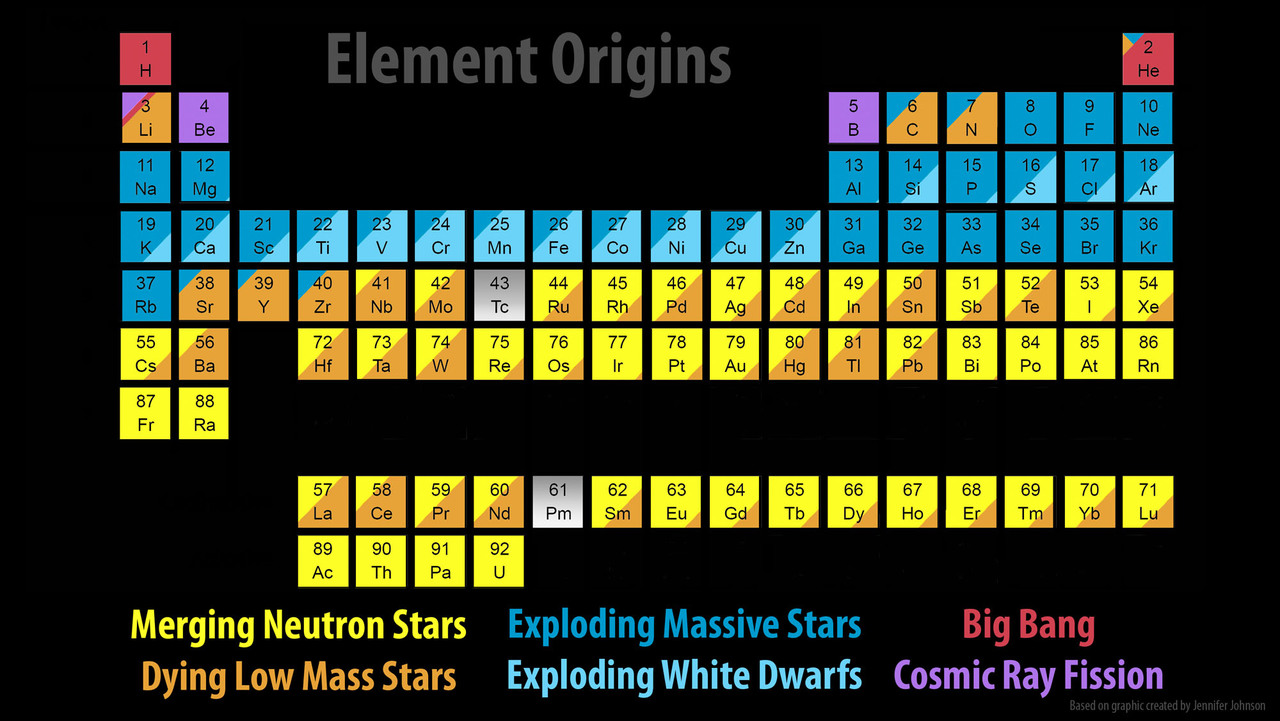
Re-writing the origin of the elements. In recent decades, astronomers have come to realize that the typical explanation for the origin of elements heavier than iron in the periodic table was not robust enough to explain the quantities of these elements we actually observe in the universe. Thanks to GW170817, we now know with great confidence that these elements (including gold and platinum) were generated during and after neutron star collisions. Credit: Jennifer Johnson/SDSS
- The nearly coincident arrival of gravitational waves and electromagnetic radiation means that we were able to constrain a distance to this event that does not rely on the cosmic distance ladder created by electromagnetic observations, which are fraught with uncertainty.

Location map of NGC4993. By Tomruen [CC BY-SA 4.0 (https://creativecommons.org/licenses/by-sa/4.0)], from Wikimedia Commons
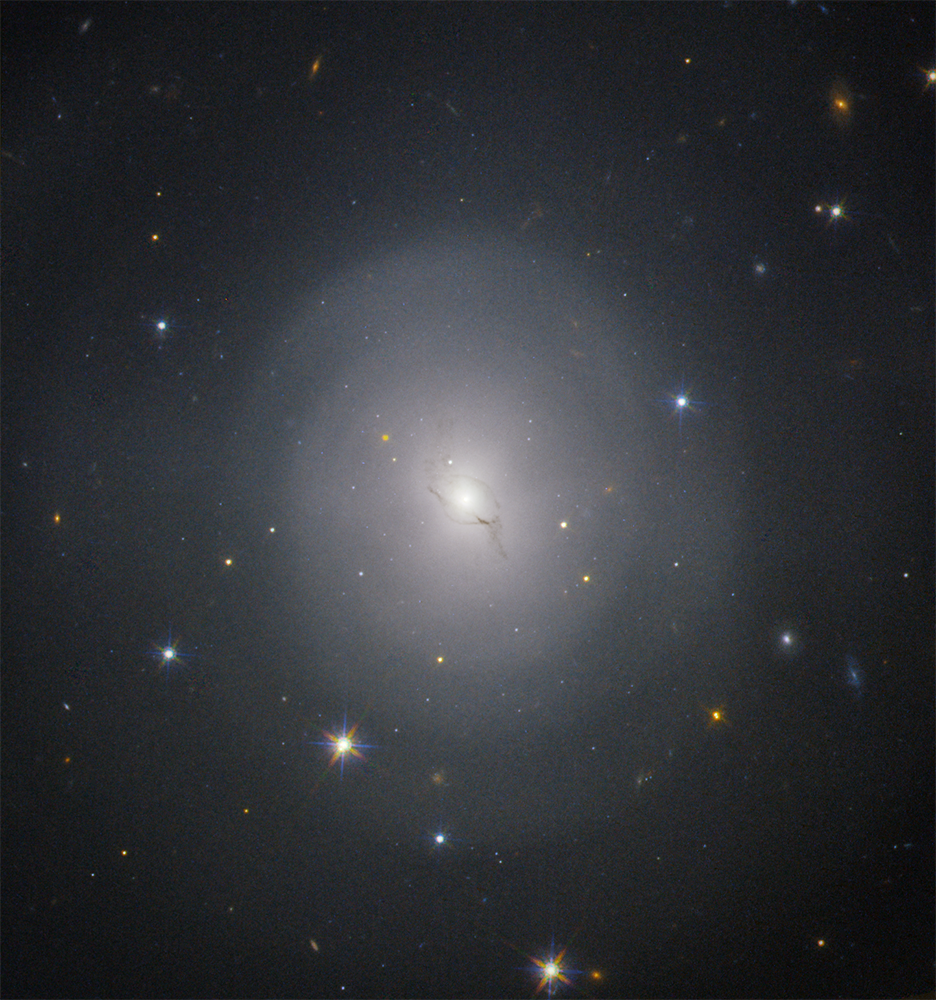
NGC4993, the galaxy that played host to the first binary neutron star merger ever detected by human beings. The galaxy is 130 million light years away from the Earth. Credit: ESA/Hubble
- The object resulting from the merger is about 2.7 times the mass of the Sun. As the object continues to be studied, evidence is mounting that what was created in the process was a low-mass black hole (if true, this would represent yet another new class of black holes discovered by LIGO). Continued observations over the next several years may confirm or refute this notion.
- Mystery of short Gamma Ray Bursts at least partly solved: With our unprecedented knowledge of the progenitor (i.e., pre-merger) masses, studies have confirmed that binary neutron star (BNS) mergers are the source of at least some (if not all) short Gamma Ray Bursts (sGRBs), a phenomenon often seen in satellite-borne detectors, but only speculated to be associated with coalescences.
- Measuring the Expansion of the Universe: With the coincident observation of GW and EM waves, we can put a new (and from an entirely different perspective) constraint on the Hubble constant, a figure which describes the rate of expansion of the universe. Results gleaned from observations of GW170817 have been consistent with pre-existing EM-based estimates. This is good news for EM astronomers who have been grappling with this issue for nearly a century.
- Gravitational waves travel at the speed of light! “Multimessenger” observations have confirmed that the speed of gravitational waves and electromagnetic (i.e., light) waves is equivalent, to a very high precision. This unequivocally confirms a basic assumption of General Relativity (the gift to science that keeps on giving!)
There’s so much more to learn about this event from further observations and through deeper study of existing observational data. Though no longer visible to optical astronomers, today, X-ray and radio astronomers are still observing the object as it evolves, and still learning about what has resulted from the collision. Doubtless, astronomers will continue to observe this object for years to come. Who knows what secrets it has yet to reveal?
Glossary
Multimessenger astronomy: Multimessenger astronomy refers to the fact that astronomers can potentially observe some events by the electromagnetic radiation (light) they emit, the gravitational waves they emit, and the particles (neutrinos or cosmic rays) they emit or generate. Each of these 'messengers' is created by a different astrophysical process, therefore revealing different information about the same source.
Neutron Star: A neutron star is the collapsed core of a large star, which before collapse, contained between 10 and 29 times the mass of the Sun. During the collapse, the star sheds much of its mass, and compacts itself into a tiny ball (tiny by cosmic standards). The resulting barren core of the progenitor star would contain between 1.4 and 2.2 times the mass of the Sun compacted into a sphere about 10 km across!