
LIGO's Interferometer

Layout of a basic Michelson interferometer with a laser as a light source. The original Michelson interferometer did not use a laser. Click to enlarge. (Credit: Caltech/MIT/LIGO Lab)
At their cores, the U.S. National Science Foundation Laser Interferometer Gravitational-wave Observatory detectors are Michelson interferometers, rudimentally similar to the sort of device that was invented in the 1880's. They are similar in that:
- They are L-shaped with two equal-length arms
- A mirror at the vertex of the arms splits a single light beam into two, directing each beam down an arm of the instrument
- Mirrors at the ends of the arms reflect the beams back to their origin point where they are recombined to create an interference pattern called 'fringes'
But this is where the similarities end. NSF LIGO’s interferometers are far larger and more complex than the builders of the world's first interferometers could have imagined.
For one thing, a big difference between the original Michelson interferometer and LIGO is that LIGO uses a laser beam as its light source, whereas the first Michelson interferometer used a plain white light.
And of course the most obvious difference between the original Michelson interferometer and LIGO's is sheer scale. With arms 4km (2.5 mi.) long, LIGO's interferometers are by far the largest ever built. (By contrast, the interferometer Michelson and Morley used in their famous experiment to study the "aether" had arms about 1.3m long). The scale of LIGO's instruments is crucial to the search for gravitational waves. The longer the arms of an interferometer, the smaller the meaurements they can make. And having to measure a change in distance 1,000 times smaller than a proton means that LIGO has to be larger and more sensitive than any interferometer ever before constructed.
While 4-km-long arms already seems enormous, if LIGO's interferometers were simple Michelson interferometers, they would still be too short to enable the detection of gravitational waves. But there are practical limitations to building a precision instrument much larger than 4km. So how can LIGO possibly make the measurements it makes?
The Longer The Better

Basic Michelson interferometer with Fabry Perot cavities. Additional mirrors are inserted near the beam splitter to facilitate multiple reflections of the laser, keeping it circulating inside the interferometer longer, and increasing the distance it travels. This greatly improves LIGO's sensitivity to the smallest changes in arm length. Click to enlarge. (Credit: Caltech/MIT/LIGO Lab)
The paradox was solved by altering the design of the Michelson interferometer to include something called "Fabry Perot cavities". The figure at left shows this modification to the basic design illustrated above. An additional mirror is placed in each arm near the beam splitter and 4km from the mirror at the end of that arm. This 4-km-long space constitutes the Fabry Perot cavity. After entering the instrument via the beam splitter, the laser in each arm bounces between these two mirrors about 300 times before being merged with the beam from the other arm. These reflections serve two functions:
1. It builds up the laser light within the interferometer, which increases LIGO's sensitivity (more photons keeping track of the lengths of the arms makes LIGO more sensitive)
2. It increases the effective distance traveled by each laser from 4km to about 1200km. (The light in Michelson's original interferometer only traveled 11 meters!) This is important because the longer the interferometer's arms, the bigger the absolute changes that gravitational waves make to total arm length (i.e., total distance traveled by the lasers). Consequently, the longer the arms and the farther the lasers travel, the bigger the effect even small gravitational waves have on the whole interferometer, making them more readily detectable.
Even with the Fabry Perot cavities, LIGO still must push the limits of what is literally physically possible in its quest to detect passing gravitational waves.
We Need More Power!
Length isn't the only limiting factor in LIGO's sensitivity. Laser power is also a consideration. Just as increasing length increases the interferometer's sensitivity to more distant gravitational waves, increasing laser power also enhances its performance. In this case, the more laser photons that merge from each arm (most photons = more power) the sharper the fringes that are measured by the photodetector.
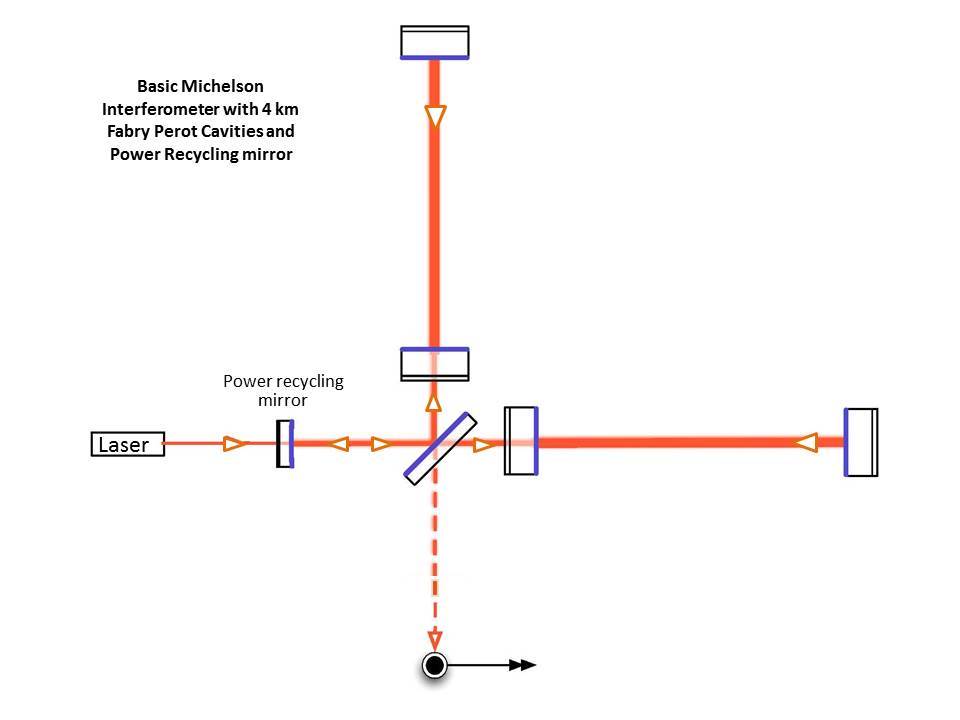
Basic Michelson with Fabry Perot cavities and Power Recycling mirror. LIGO's interferometers use multiple power recycling mirrors but for simplicity only one is shown. Click to enlarge. (Credit: Caltech/MIT/LIGO Lab)
But there's a problem here too. LIGO's laser first enters the interferometer with a power of about 40 Watts, but it needs to operate closer to 750kW if it has any hope of detecting gravitational waves. Here we have another paradox. Just as it would be impossible to build a 1200km-long interferometer, building a laser with this initial power is a practical impossibility.
Once again, LIGO uses mirrors to solve this dilemma. They are called Power Recycling Mirrors. The figure at left shows schematically where a power recycling mirror is located inside each interferometer.
Inside the interferometer, light from the laser passes through the transparent side of a power recycling mirror to the beam splitter where it is directed down the arms of the interferometer. The instrument's alignment and mirror coatings, and even quantum mechanics, ensure that nearly all of the laser light entering the arms follows a path back to the reflective side of the power recycling mirror before it exits to the photodetector. As laser power is constantly entering the interferometer from the laser itself, the power recycling mirror continually reflects the laser light that has already traveled through the instrument back into the interferometer (hence 'recycling'). This process greatly boosts the power of the laser light 'stored' inside the Fabry Perot cavities without the need to generate such a powerful laser beam at the outset.
The boost in power generated by power recycling results in a sharpening of the interference fringes that appear when the two beams are superimposed--fringes which will tell scientists if a gravitational wave has passed. The sharper the fringes, the easier it becomes to identify the tell-tale signs of gravitational waves.
Other Beneficial Features
Two other modifications make LIGO's interferometers unique and able to make the world's smallest measurements. First, they also possess signal recycling mirrors, which further enhance the signal that is received by the photodetector. And second, LIGO's interferometers were constructed with extraordinary mechanisms to damp out unwanted vibrations (noise) making it easier for the instruments to sense just the vibrations caused by gravitational waves. LIGO's seismic isolation system is discussed in much greater detail in LIGO Technology.
With these modifications, LIGO's interferometers are more precisely known as a dual recycled, Fabry-Perot, Michelson interferometers.