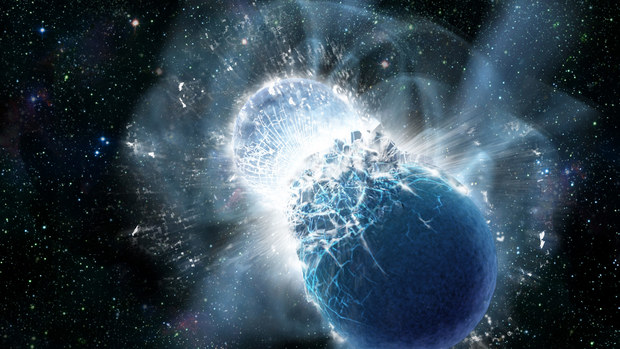
Artist's depiction of a neutron star collision after inspiral. (Credit: NASA/Swift/Dana Berry)
LIGO Detection of Colliding Neutron Stars Spawns Global Effort to Study the Rare Event
News Release • October 16, 2017
By Kimberly Burtnyk
[Click HERE to read the official LIGO Scientific Collaboration/LIGO Laboratory press release]
LIGO’s latest gravitational wave detection has spawned an explosion of new science across the global astronomical community. On August 17, 2017, the two LIGO instruments (funded by the National Science Foundation) and its sister facility, Virgo, near Pisa, Italy, sensed tell-tale signs of the remnant cores of two massive stars spiraling toward and then smashing into each other some 130 million light years away. The objects were quickly identified as neutron stars, the collapsed cores of stars that were once much more massive than our Sun. They are called “neutron stars” because their matter is so densely packed it is composed primarily of neutrons. One such star containing as much matter as our Sun would be just 10 to 15 km in diameter, and a teaspoon of its material would weigh about one-billion tons on Earth. Using the signals received in LIGO’s detectors, the masses of the neutron stars were determined to 1.1 to 1.6 times as massive as our Sun.
LIGO Hanford Observatory (LHO) Head, Michael Landry explained what LIGO saw when it made this landmark discovery:
“LIGO and Virgo detected 100 seconds of gravitational waves as these two neutron stars spiraled together in a massive and fiery collision,” he said. “In a sprawling follow-up campaign involving about one-quarter of the world’s professional astronomers, observatories in space and on the Earth have detected radiation in all wavelengths from gamma rays to radio waves. But the LIGO and Virgo detectors were absolutely essential in identifying and pinpointing the event in the sky, allowing this campaign to proceed”, Landry added.
This discovery adds a new way of learning about the universe through “multi-messenger astronomy”, where data from traditional telescopes, neutrino detectors, and now gravitational wave observatories are shared and compared to glean even deeper insights into the nature of the universe.
When It Rains, It Pours
This historic detection came just three days after another historic detection, LIGO’s fourth, which was also detected by the Virgo interferometer in Pisa Italy, making it the first detection by Virgo, and the first three-detector observation of a gravitational wave. Reveling after that event, LIGO scientists were astonished to learn of yet another detection, this one completely different from anything LIGO had seen before.
Salvatore (Salvo) Vitale, assistant professor of physics at MIT, was attending a conference in Amsterdam along with other LIGO scientists, when he first got word of this second detection in 3 days. The first alert he received included a ‘false alarm rate’ (FAR), a measure of how likely it is that the event was not real. In this case, the FAR was reported as 3x10-12, which is, according to Vitale, “ridiculously low!”
How ridiculously low? This figure suggests that the chance that some random but nearly identical bits of ‘noise’ that happened to look like a gravitational waves appeared in the instruments at essentially the same time was less than 1 in 80,000 years.
Two minutes after that first alert, the first scan of the event, automatically generated from the Hanford data, was distributed, and it was distinctly different from anything LIGO had seen before. Signals of black hole mergers last just fractions of a second. This signal lasted well in excess of 30 seconds (in the end, it was shown to have lasted nearly 2 minutes, 500 times longer than black hole mergers). This was a clear indicator that the objects that created the signal were much less massive than black holes. To Vitale and everyone else, the unique properties of the signal could mean only one thing: LIGO had caught its first gravitational wave from merging neutron stars.
This was in itself a surprise, as Vitale explained. “I saw the omega scan from Hanford, and saw that there was a clear chirp signal, which I remember thinking is ridiculous, because we never thought we’d see anything in an omega scan from a binary neutron star merger … But this [one] was so loud that we saw it too!”

Top: Thirty-seconds worth of binary neutron star inspiral as it appeared in the LIGO detectors. The entire signal lasted 100 seconds. Bottom: LIGO's first black hole merger detection. The duration of the "chirp" was just 0.2 seconds; 500 times shorter than the signal generated by the neutron stars. (Caltech/MIT/LIGO Lab)
At the same time that all this was happening, LIGO scientists were alerted to another remarkable astronomical event, which occurred within 2 seconds of LIGO’s detection. The Fermi gamma ray space telescope had recorded a "short" gamma ray burst (sGRB) just 1.7 seconds after the arrival of the gravitational waves.
Gamma ray bursts are seen quite frequently, but what causes them has remained a mystery. Knowing that neutron star mergers were expected to generate electromagnetic radiation, likely of very high energy, excitement among LIGO scientists began to grow as it became more and more plausible that the first electromagnetic counterpart to a gravitational wave (GW) had been observed. The time of arrival of the sGRB and GW signals was especially telling, and important to validating the relationship between them.
Vitale explained, “You want the gamma ray burst to come after the gravitational waves because first you have to smash the objects together, then the material is warmed up, and then you get the radiation. So you would expect to see the gravitational waves first.”
As the pieces began to fall into place, the magnitude of LIGO’s detection became all the more weighty.
“Then it was, like… ‘Okay. Oookay…let’s take a chair… and sit down…” said Vitale, laughing as he recalled his feelings at that moment.
The only way to confirm a correlation between the GRB and the GW, however, would be to find the source object on the sky; but there was a problem. At that point, only the LIGO Hanford data had been processed and distributed; without the Livingston data, no such localization of a source would be possible.
Matt Evans (Assistant Professor of Physics at MIT) recalls the flurry of communication he was receiving in those early moments.
“There was this hubbub by Salvo talking about a signal at LHO that looked like a binary neutron star coincident with the Fermi alert. But there hadn’t been anything from Livingston, so there was a moment of doubt of the validity of the signal.”

LSC scientist and University of Chicago Kavli Institute Postdoc, Reed Essick, can barely contain his excitement while visiting the KAGRA gravitational wave observatory in Japan. (Image: Reed Essick, with permission)
The missing data from Livingston was puzzling. Reed Essick (Postdoctoral Fellow, UChicago Kavli Institute for Cosmological Physics) explained:
"On the search side, everything looked good, and a sanity check of the detectors told us that LLO was in science-mode. So why didn’t the event ‘trigger’ in Livingston?”
Essick decided to check Livingston data for ‘glitches’, random bits of loud, sudden noise that can drown out other signals in the detectors. Running an algorithm designed specifically for this task, Essick saw that a glitch had in fact occurred at LLO at the same time that the signal appeared in the Hanford interferometer. Sifting through the files manually, Essick found the data stamped with the time of the glitch (and the detection), and there it was.
This was why LLO didn’t automatically send out a trigger alert. The glitch caused LLO’s computers to disregard, or ‘veto’ that part of the data stream. Looking at it, it’s no wonder! At first glance, it looked ugly. However, Evans explained that it really wasn’t as bad as it seemed.
“The glitch looks really terrible on the scan. But the truth is, it’s large in amplitude and short in time, so it wouldn’t ruin our ability to do any science on it.”
Evans added, “Glitches happen every few hours, so the probability of one landing on top of a signal is very low. Nevertheless, people have been working on this sort of possibility for a while, so we were prepared.”

The glitch that prevented Livingston's automated system from distributing the signal from the merging neutron stars. Despite the glitch, the curved GW signal is clearly visible. It looked bad at first, but only 10 ms of data needed to be cleaned up.
Most remarkably, despite the size of the glitch, the gravitational wave signal itself was still clearly visible (see image at right). Seeing that was a moment that Vitale remembered vividly.
“It was a mix of happiness, tension, and disbelief. We saw that beautiful image of the chirp going through the glitch and coming out the other side. And at that point, it was pretty incredible.”
In a mathematical equivalent of a game of Operation, at least three teams of people began working on separating the glitch from the signal. As with everything LIGO-related, even cleaning a fraction of a second of data required a group effort! Ultimately, the work paid off. After a few hours, the glitch had been cleanly removed, and some extraordinary science was about to begin.
Spreading the Word
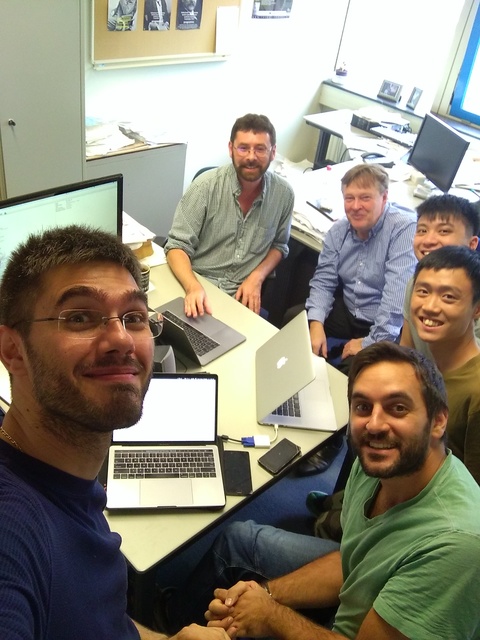
"The six happiest people in the world" on August 17, 2017. Salvatore Vitale (left) snapped this photo moments after first seeing the LLO scan. The tell-tale trace of gravitational waves generated by merging neutron stars was clearly visible. (Clockwise from top: Chris van den Broeck, Jo van den Brand, Peter Pang, Ka Wa Tsang, and Michalis Agathos. Image: Salvo Vitale, with permission.)
In anticipation of this kind of event, LIGO has signed agreements with 90 astronomical observatories around the world to hunt for signs of electromagnetic (EM) radiation escaping from a gravitational wave event. LIGO shares sky-coordinates with its EM partners, who can then start searching for an EM counterpart. Until August 17th, 2017, no one had found any such counterpart, but the lure of being among the first ones to detect EM radiation from a gravitational wave event has kept LIGO’s astronomy partners engaged for over 10 years. This event, combined with a coincident gamma ray detection, represented the best chance yet for astronomers to find something, but to do so, LIGO had to tell them where to look. To that end, LLO data (cleaned of the glitch) were combined with Hanford and Virgo data, and a sky-map narrowing down the possible location of the source of the gravitational waves was generated. The GW map was then overlaid on Fermi’s GRB map and another region calculated using the INTEGRAL gamma ray space telescope. The result was nothing short of amazing.
The location of the source of the gravitational waves as predicted by LIGO-Virgo data sat beautifully inside the regions of the sky estimated to contain the source of the gamma ray burst as determined by Fermi and INTEGRAL. The resulting search area was small enough that within 12 hours LIGO’s optical astronomy partners had successfully tracked down and imaged a residual fireball at the edge of a galaxy (NGC4993) some 130 million light years distant–most of that time was spent waiting for dusk in Chile, where the first observations could be made. The long-awaited discovery of an EM counterpart to a gravitational-wave detection had been confirmed! Furthermore, the answer to another long-sought-after question was solved: Astronomers could now say with certainty, that at least one source of short gamma ray bursts in the universe is merging neutron stars.
The skymap created by LIGO-Virgo (green) showing the possible location of the source of gravitational waves, compared with regions containing the location of the gamma ray burst source from Fermi (purple) and INTEGRAL (grey). The inset shows the actual position of the galaxy (orange star) containing the "optical transient" that resulted from the merger of two neutron stars (Credit: NASA/ESO)

The first image of an 'optical transient' resulting from the merger of two neutron stars, and the first image of an optical counterpart to a gravitational wave detection. The box at left shows the host galaxy NGC4993, 130 million light years distant, as it appeared in a Hubble Space Telescope image taken April 28, 2017. At right is the same galaxy imaged by the Swope Telescope just a few hours after the gravitational wave and gamma ray detections on August 17, 2017. The arrow points to the short-lived visible fireball that resulted from the merger of two neutron stars in that galaxy. (Credit: Swope Supernova Survey via UC Santa Cruz).
For LIGO, this optical observation was important for another reason. The distance to the galaxy, as determined by astronomers, was wholly consistent with LIGO’s estimated distance of the source of the gravitational waves. Thus, the astronomers provided a completely independent verification that LIGO’s methods for determining the distances to gravitational wave sources are sound.
From that moment on, all eyes were, and continue to be (now two months after the initial detection), on the skies. LIGOs astronomy partners immediately began observing this object in every wavelength possible, from gamma rays to visible radiation to radio waves, as the remnant ‘object’ changes over time. To date, some 70 of LIGO’s optical astronomy partners have observed this extraordinary event.
Today, few would disagree with the statement that the level of interest in and study of this event is unprecedented. Within 8 weeks of the detection, over 100 scientific papers describing the results of follow-up studies were written by scientists around the world. Dozens of these papers were published on Monday, October 16th alone, with many more certain to follow in the months and possibly years to come, making this the most broadly and intensely studied astronomical event in human history.
Deeper Meaning
Beyond the obvious scientific importance of this discovery, the importance of this event for the LIGO Laboratory and the wider collaboration goes much deeper. For many, this single detection represents the apex of careers, the culmination of decades of hard work and dedication to LIGO and gravitational wave science.
Dr. Fred Raab, Associate Director for Observatory Operations at the LIGO Hanford Observatory, shared what this latest discovery means to him:
“After nearly 30 years of working toward this discovery, I knew that observing the last minute of a binary neutron star system would give unprecedented precision in its parameters. Yet I was unable to continue reading an early paper draft past where I first saw the number for the chirp mass, a key parameter of the system. I stared in wonder for minutes at that number, measured to 4 significant figures for a pair of stars more than 100 million light years from Earth.”
Raab continued, “This observation means that LIGO is transitioning now from studying extreme regions of space-time to extreme states of matter.”
Mike Zucker, LIGO Systems Scientist, had a similar reaction in those first days after the detection:
“I literally stayed awake for days after GW170817 watching the [astronomy notices] roll in, marveling at all the extraordinary implications as the revelations topped each other one by one. I’m just a detector mechanic, but I consider this to be the most significant achievement of my career.”
Janeen Romie, LLO’s Detector Engineering Group Lead, was in her office in Livingston talking to her husband on the phone when she got the first alert:
"I noticed that it was a binary neutron star merger and I was like, ‘I’ve got to get off the phone! I’ve got to run down the hall! I’ve got to find out what’s going on!"
Unable to share the news with anyone outside of LIGO, the only thing Romie could do is run and talk to her colleagues.
"That’s why it was so funny for me,” she laughed, “I hung up on my husband!”
Matt Evans shared what he believes is most meaningful to the LIGO laboratory in light of this discovery:
“This detection, and especially the triple binary black hole detection with Virgo are important because they demonstrate that we (LIGO) are not the only ones claiming to detect gravitational waves”, he said. “This event solidifies our position in astronomy, not just physics. Other projects around the world will benefit greatly. We really can do multi-messenger astronomy, and that is really meaningful and useful.”
This sentiment was echoed by Essick and Vitale:
“What’s most important is represented by the ‘O’ in LIGO”, said Essick, referring to the fact that the “O” in LIGO stands for Observatory. “We’ve been selling the idea that we will detect binary neutron stars for decades, and now we’ve finally done it. We’ve delivered on that promise.”
Vitale would agree, “I think this event brings us a step closer to astronomy", he said. "The detection of these events… it’s not just ‘collecting stamps’ anymore. Now we can do lots of cool stuff.”
Salvatore's feelings ran a bit deeper still:
“Those few days were among the most beautiful days of my life”, he said. “We kept receiving the circulars from the astronomers. They’d say, ‘oh, we have a GRB’, ‘oh, we have found an EM counterpart in optical’, ‘oh, we found the galaxy’, ‘we found the x-rays’, etc. It was… incredible.”
He paused for a moment; then continued:
“It’s also been sad”, he said. “I don’t know if I’ll ever live a moment like that again in my life.”
It's doubtful that anyone at LIGO will.